Invasive shrubs are flourishing in temperate, deciduous forest understories of eastern North America where resources, especially light, are limited. However, understory light is more available before the overstory canopy leaves emerge in the spring and after fall senescence. Extended leaf phenology of invasive shrubs in the spring and fall compared to native shrubs and the overstory canopy is conspicuous, as well as higher foliage abundance of invasive compared to native shrubs. Extended leaf phenology of invasive shrubs provides photosynthetic benefits, but also seasonally novel shade. Light and temperature regulate life history characteristics across taxa and influence ecosystem processes. Here, a long-term invasive shrub removal experiment is used to quantify the effect on light and air temperature over 3–4 y. There was a pattern of reduced maximum air temperature in the presence of invasive shrubs during the growing season of most years. We find less light energy infiltration (lumens m–2) below invasive shrubs than native shrubs with mean differences largest in the spring (–1981 [–2604, –1380], a 26.8% reduction relative to below natives). Differences diminish through summer (–1038 [–1221, –845]), fall (–547 [–660, –429]), and winter (–257 [–372, –151]). Invasive shrubs also filter more photosynthetically active radiation than natives (58.4 [38.5, 78.7] µmol m–2 s–1, a 39.4% reduction), but seasonal differences were not detected indicating denser canopy structure for invasive shrubs throughout the year. When compared to the native understory community, the presence of invasive shrubs seasonally reduces temperature and light availability near the forest floor, which could affect resident plant and animal species.
How to translate text using browser tools
2 August 2021
The Seasonal Influence of Invasive Shrubs on Light and Temperature in an Eastern Deciduous Forest Understory
Erynn Maynard-Bean,
Margot Kaye
ACCESS THE FULL ARTICLE
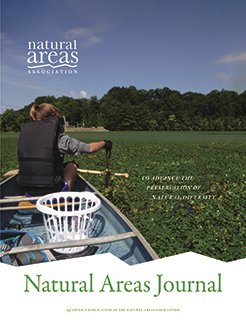
Natural Areas Journal
Vol. 41 • No. 3
July 2021
Vol. 41 • No. 3
July 2021
central Appalachian hardwood
extended leaf phenology
invasive shrubs
lumens
novel shade
phenology
photosynthetically active radiation