The ichnospecies Lunulipes obscurus is reevaluated and a new diagnosis provided. All samples from Early Jurassic lacustrine deposits of the Deerfield Basin in Massachusetts, USA that were previously determined to bear Lunulipes or Lunulipes-like traces were examined. This reevaluation revealed that the ichnospecies is restricted only to two slabs. The morphology of Lunulipes obscurus suggests that its arthropod maker beat a single pair of legs synchronously. This type of locomotion is used by aquatic insects such as backswimmers (Notonectidae), water boatmen (Corixidae), and predaceous diving beetles (Dytiscidae). Neoichnological experiments with these three taxa indicate that water boatmen produce trackways that are the most similar to the fossil, and hence we conclude that a water boatman or an unknown insect that employed a similar method of swimming produced the Early Jurassic traces. These trackways provide the first tentative evidence of water boatmen in the Deerfield Basin, and, if correctly identified, represent the first trace fossils of this aquatic insect group anywhere.
How to translate text using browser tools
20 April 2018
Aquatic insect trackways from Jurassic playa lakes: Reinterpretation of Lunulipes obscurus (Hitchcock, 1865) based on neoichnological experiments
Patrick R. Getty,
Samuel B. Loeb
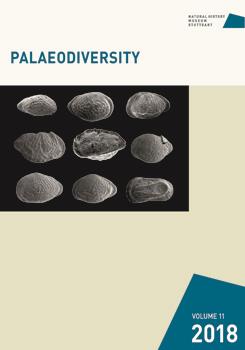
Palaeodiversity
Vol. 11 • No. 1
December 2018
Vol. 11 • No. 1
December 2018
Actuopaleontology
Corixidae
Dytiscidae
Early Jurassic
ichnology